In the past couple of weeks I’ve read two things I want to recommend that are actually deeply related. The first is Brian Potter’s excellent history of technology analysis of the evolution of the lithium ion battery. Brian’s Construction Physics newsletter is a consistently excellent treasure trove of analysis, from his series on the history of the electricity industry (Part I)(Part II)(Part III)(Part IV) to his retrospective on Bell Labs. [note: as it happens, both of the authors I’ve been reading are named Brian, so I’m reverting to formal last names for reference although I know both personally.]
The history of lithium ion battery that Potter tells has origins in Alessandro Volta’s “pile” battery in 1800, with a long tail of experimentation for the next 160 years. In the 1960s liquid electrodes were developed in an industrial lab at Ford, demonstrating that a phenomenon called fast ion transport exists. Subsequent academic research at Stanford explored the why and how of the phenomenon, and discovering a new-to-batteries phenomenon, intercalation (electrons moving in and out of solid layers in an electrode). Then research in an industrial lab at Exxon focused on creating intercalation and fast ion transport in other battery chemistries and configurations. After that, in the 1970s-1980s, a lot more targeted academic and industry research worked to develop cathodes that were safer and had durable materials that didn’t degrade with discharging and recharging, and to find substitutes for the volatile lithium in the anode. Note that this evolution is also a very international development, from the US to the UK to France to Japan, in an open community of theoretical and applied science. Finally, Sony slowly, methodically, developed a commercializable product in 1991, and subsequent development has continued, detailed brilliantly in Potter’s essay. If you have not read it yet, please go do so now!
Combinatorial Innovation and Complexity Science
The other (re-)reading I’ve been doing is Brian Arthur’s The Nature of Technology (2009). Arthur presents a theory of combinatorial innovation, which he views as the foundation of technological evolution. Arthur argues that technologies are not created ex nihilobut emerge through the recombination of existing components and ideas. This concept, rooted in complexity science, offers insights into how innovation occurs, evolves, and reshapes our world and our capabilities.
Arthur’s core insight is that technologies are constructed from existing technologies, and that these technologies evolve through organic processes of combination, recombination, and withering of technologies made obsolete by new recombinations. Technologies are inherently modular systems, composed of distinct building blocks or sub-technologies. These components can be combined, recombined, and improved upon to create new technologies or enhance existing ones.
Combinatorial innovation as a theory of technological change has distinctive elements, with the characteristic element being recursive building: new technologies become building blocks for future innovations. For example, the development of microchips enabled personal computers, which in turn facilitated the creation of the internet and countless digital applications. Another is the emergence of novelty, because the interaction of components can lead to emergent properties, producing technologies with capabilities far beyond the sum of their parts. Emergent novelty is why breakthroughs often occur at the intersections of different domains, such as biotechnology blending genetics and computing. It’s also why so many new discoveries are serendipitous and unintended, such as the well-known story of the 3M researcher discovering the adhesive for Post-It Notes while working on something completely different. Finally, Arthur situates this theory squarely in evolutionary theory. Combinatorial innovation mirrors biological evolution, where variation, selection, and recombination drive complexity and adaptation. Technologies evolve not only by improving individual components but also by rearranging and reconfiguring them in response to new challenges or opportunities.
Arthur’s ideas on combinatorial innovation draw heavily from complexity science, a field that studies how interactions among simple elements give rise to complex systems. Several principles of complexity science underpin his framework, most notably the aforementioned emergent behavior. Just as ecosystems or markets exhibit emergent properties, technological systems produce novel behaviors through the interplay of components. For example, combining GPS and cellular networks enabled real-time navigation, an emergent capability not foreseen in their original design. Another insight from complexity science is path dependence; technological evolution, like other complex systems, is highly path-dependent. The historical availability of certain components and ideas shapes the trajectory of innovation. For example, the dominance of internal combustion engines influenced infrastructure and delayed the adoption of electric vehicles. Networks and interconnectivity are another foundational concept, and combinatorial innovation incorporates the idea that technologies evolve within networks of knowledge, where components and ideas interact dynamically and can ripple across different fields of expertise. Finally, feedback loops, a hallmark of complex systems, play a central role in combinatorial innovation. For instance, the success of digital technologies spurred investment in microelectronics, creating a self-reinforcing cycle of improvement and adoption.
Lithium Ion Batteries as Combinatorial Innovation
Potter’s telling of the technology history of the lithium ion battery illustrates the extent to which it was a process of combinatorial innovation. Lithium-ion battery technology exemplifies recursive building, where new innovations build upon prior advancements, turning technologies into components for subsequent generations. The battery’s foundational chemistry, rooted in the study of lithium as a lightweight, high-energy material, was first explored in the 1970s. Early designs used lithium metal anodes, but stability and safety challenges necessitated refinements, leading to the adoption of intercalation chemistry. These advances were not endpoints but stepping stones. The development of lithium cobalt oxide cathodes and graphite anodes in the 1980s provided the structural and electrochemical reliability needed for commercial success. Once commercialized by Sony in 1991, lithium-ion batteries became building blocks for new innovations, powering laptops, smartphones, and, eventually, electric vehicles. As each iteration of the technology refined its components, these improvements created platforms for broader applications, enabling recursive cycles of innovation.
The evolution of the lithium-ion battery also underscores the emergence of novelty through the recombination of ideas. Technologies rarely progress in linear, predictable ways; rather, breakthroughs arise from the intersection of diverse disciplines. For lithium-ion batteries, this process involved the integration of electrochemistry, materials science, and systems engineering. Safety mechanisms, such as separators and thermal management systems, were developed by combining insights from polymer science and manufacturing engineering. Similarly, advances in energy density required innovations not just in materials but also in the electronics that manage charge and discharge cycles. These emergent capabilities illustrate how combining elements in new configurations leads to outcomes that transcend the sum of their parts, such as the ability to power vehicles or stabilize electricity grids.
The trajectory of lithium-ion technology is best understood as an evolutionary process (and still in process right now), shaped by variation, selection, and recombination. Early designs were constrained by their reliance on scarce or expensive materials and their limited energy density. Market forces and user demands acted as selective pressures, favoring iterations that addressed these limitations. New chemistries, such as nickel-manganese-cobalt and lithium iron phosphate, were introduced to balance cost, safety, and performance, adapting to changing market and regulatory environments. Like biological evolution, the development of lithium-ion batteries has been path-dependent, influenced by historical choices and constraints. For instance, the early dominance of lithium-ion chemistry shaped infrastructure and research priorities, creating barriers for competing technologies like solid-state or sodium-ion batteries. This evolutionary dynamic illustrates how technologies adapt within specific contexts, but also how their trajectory is a function of past developments.
The lens of complexity science enriches this understanding of lithium-ion battery innovation. One key insight is the role of emergent behavior in technological systems. When lithium-ion batteries were first introduced, their potential applications were not fully understood. Over time, their integration into smartphones, laptops, and electric vehicles created cascading effects across industries, from transportation to telecommunications. The interaction of these applications, in turn, spurred further demand for energy-dense, long-lasting batteries, creating feedback loops that accelerated innovation. These emergent dynamics reflect the complexity of technological ecosystems, where advances in one domain ripple across others.
Path dependence, another concept from complexity science, highlights how initial conditions shape the trajectory of innovation. The early investment in lithium-ion chemistry, catalyzed by Sony’s decision to commercialize it for consumer electronics, created a self-reinforcing cycle of research and development. This trajectory led to the refinement of manufacturing processes, economies of scale, and infrastructure, such as supply chains for lithium and cobalt. These historical choices locked in certain pathways, making it more challenging for alternative technologies to compete, even as lithium-ion batteries faced environmental and resource constraints.
The networked nature of innovation further amplifies the role of combinatorial creativity. Lithium-ion technology evolved within an international network of knowledge exchange involving universities, corporations, and governments. Collaborative research initiatives, such as partnerships between automakers and battery manufacturers, enabled breakthroughs in performance and cost reduction. Knowledge spillovers between industries—such as the application of semiconductor manufacturing techniques to battery production, and more recently the use of AI to identify new battery chemistries more quickly—illustrate how interconnected systems drive progress. These networks of collaboration and competition demonstrate how innovation thrives in environments where ideas and expertise circulate freely.
Finally, feedback loops play a central role in the lithium-ion battery’s rapid cost decline and widespread adoption. As cumulative production increased, manufacturing efficiencies improved, and economies of scale reduced costs. These cost reductions, in turn, spurred further investment and expanded markets, reinforcing the learning curve dynamic. Each doubling of production brought measurable cost declines, estimated at 18-20%. This iterative cycle demonstrates how feedback loops, a hallmark of complexity, drive exponential progress in technology.
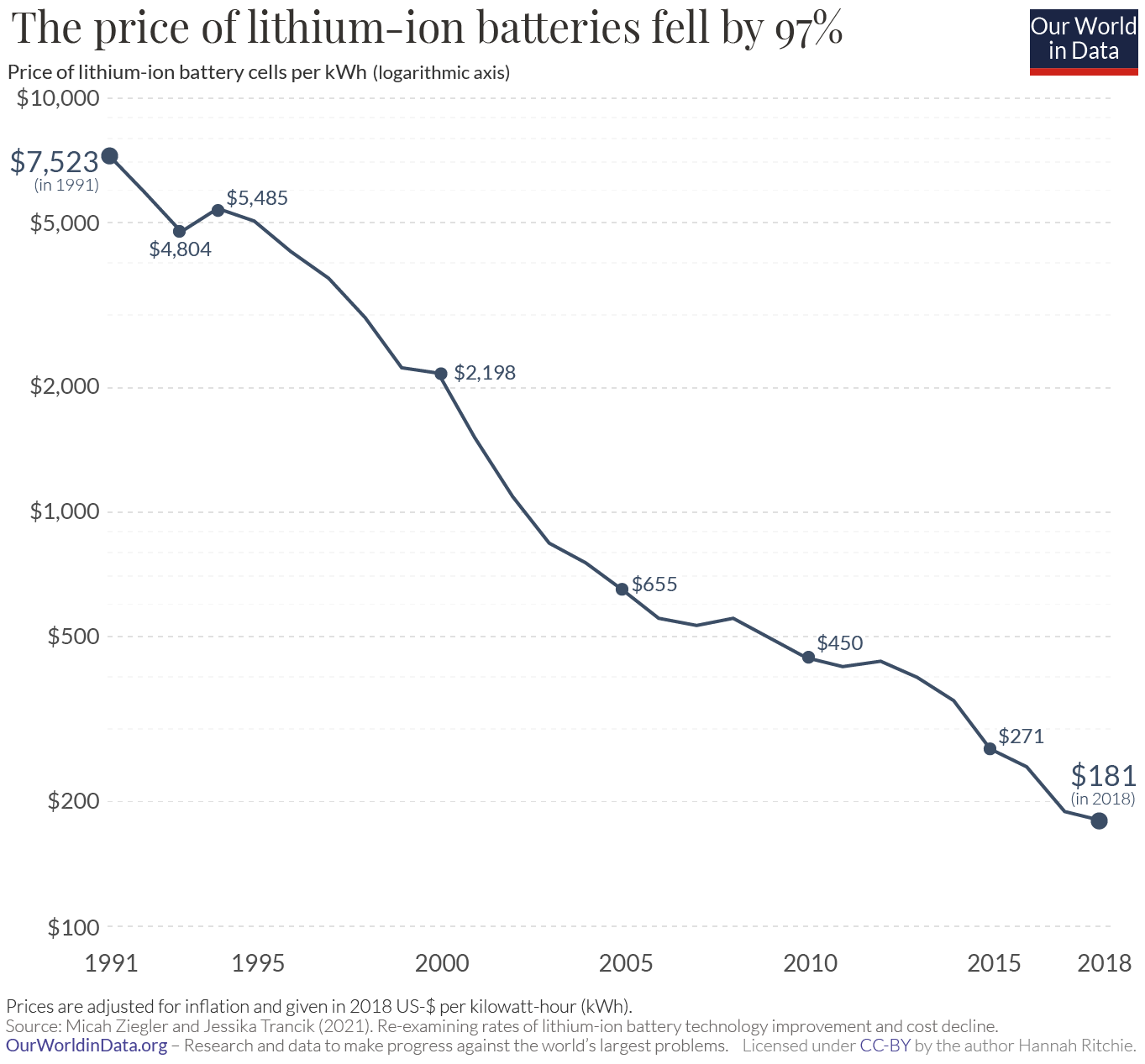
The story of the lithium-ion battery’s evolution that Brian Potter told so well thus represents a confluence of combinatorial innovation and complexity science. It is a story of recursive building, where each innovation becomes a foundation for the next; the emergence of novelty, as interdisciplinary ideas combine to create new possibilities; and evolutionary processes, shaped by adaptation, selection, and path dependence. The principles of complexity science—emergent behavior, networks, and feedback loops—offer a powerful framework for understanding how these dynamics unfold in technological ecosystems.
This post was originally published on Lynne’s Substack, Knowlegde Problem. If you enjoyed this piece, please consider subscribing here.